Revolutionizing Technology: Advancing Next-Gen Sequencing
Discover the possibilities of next generation and third generation sequencing technology. Revolutionize your research with our advanced approach.
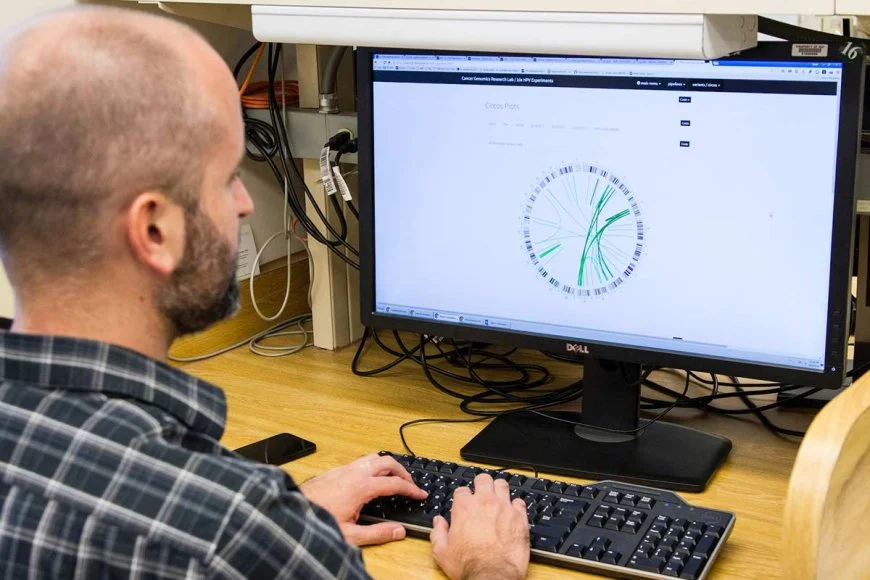
Are you curious about the incredible possibilities that can be achieved with next-generation sequencing technologies like Novaseq? These advanced techniques, such as the ones used in the Human Genome Project, have revolutionized DNA sequencing, allowing us to uncover the mysteries hidden within our human genomes. With next-generation sequencing (NGS), millions of DNA fragments can be sequenced simultaneously, providing high-throughput capabilities like never before. On the other hand, third-generation sequencing offers long-read capabilities, enabling us to analyze complex genomic regions that were previously challenging to decipher. From new generation platforms to proton sequencing and protein nanopore techniques, these sequencing technologies have transformed the field of genomics. Get ready to dive into the world of cutting-edge sequence data analysis and explore the fascinating possibilities that arise from these remarkable advancements in technology.
So, what exactly can we achieve with these Novaseq and third-generation sequencing technologies? Let's delve deeper into this exciting realm of NGS and explore their applications in the Human Genome Project and the study of complex genomes!
Exploring Next-Generation Sequencing (NGS)
Next-generation sequencing (NGS), including platforms like Illumina's NovaSeq, has revolutionized genomics by enabling scientists to study complex genomes and gain valuable insights. These advancements in technology have made NGS a powerful tool for sequencing human genomes. Let's explore the possibilities offered by these next-generation sequencing technologies, along with the informatics involved in analyzing the data.
Accurate Identification of Genetic Variations
One of the key advantages of next generation sequencing (NGS) is its ability to accurately identify genetic variations, including single nucleotide polymorphisms (SNPs). SNPs are variations in a single nucleotide base pair that can have significant implications on an individual's susceptibility to diseases or response to medications. By leveraging NGS platforms, researchers can efficiently analyze large volumes of genetic data and pinpoint these crucial variations with precision. This makes NGS a valuable tool for RNA sequencing, cell sequencing, and whole genome sequencing.
Unveiling Gene Expression Patterns through RNA-seq Analysis
NGS, or next-generation sequencing, also enables researchers to study gene expression patterns through RNA-seq analysis. This technique allows scientists to examine which genes are active in specific tissues or under certain conditions. By sequencing RNA molecules derived from cellular samples, NGS provides a comprehensive snapshot of gene activity within an organism, including the human genome. This information is invaluable for understanding biological processes, identifying disease mechanisms, and developing targeted therapies using the novaseq platform.
Advancements in Nanopore Sequencing
Nanopore sequencing, an emerging technology within the realm of Next Generation Sequencing (NGS), holds immense promise. It utilizes nanopores—tiny holes that allow individual DNA strands to pass through—to directly read DNA sequences. Oxford Nanopore Technologies is at the forefront of this innovation, offering portable sequencers capable of real-time DNA analysis. The portability and speed of nanopore sequencing make it a compelling option for field research, infectious disease surveillance, rapid diagnostics, and gene panels. With the ability to analyze large amounts of nucleotides quickly, nanopore sequencing is a game-changer in genomics. It offers an alternative to traditional Sanger sequencing methods.
Exploring Epigenetic Sequencing
Epigenetic modifications play a critical role in regulating gene expression and can have a profound impact on an individual's health and development. NGS, or next-generation sequencing, has facilitated the exploration of epigenetic sequencing, allowing scientists to unravel the complex interplay between genetic and environmental factors. By mapping DNA methylation patterns or profiling histone modifications, researchers gain insights into how epigenetic changes influence disease susceptibility, aging processes, behavior, and the human genome. Whole genome sequencing using technologies like NovaSeq has enabled the comprehensive analysis of nucleotides to further understand these epigenetic mechanisms.
Complementing Traditional Sanger Sequencing
While Next Generation Sequencing (NGS) has emerged as a powerful tool, it does not render traditional Sanger sequencing obsolete. Instead, these two approaches, NGS and Sanger sequencing, complement each other in different contexts. Sanger sequencing remains the gold standard for validating NGS results and resolving complex genomic regions. Its accuracy and ability to sequence long DNA fragments make it indispensable for certain applications such as studying structural variations or confirming rare variants identified by NGS. This is particularly relevant when using advanced technologies like NovaSeq and gene panels for whole genome sequencing. By utilizing SGS technologies, researchers can harness the combined power of NGS and Sanger sequencing to gain comprehensive insights into the intricacies of the genome.
Advancements in Third-Generation Sequencing Technology
Third-generation sequencing platforms, such as PacBio and Oxford Nanopore, have revolutionized the field of DNA sequencing by offering longer read lengths compared to traditional Next-Generation Sequencing (NGS) methods. These technologies utilize single-molecule sequencing methods, including polymerase chain reaction (PCR) and Sanger sequencing, which involve the use of nucleotides for determining the sequence of DNA. These methods provide several advantages over NGS approaches.
One significant advantage of third-generation sequencing is the ability to generate longer DNA sequences. With NGS, read lengths are typically limited to a few hundred nucleotides. In contrast, third-generation sequencing platforms can produce reads spanning thousands of nucleotides. This extended read length enables researchers to assemble complex genomes more accurately and efficiently due to the epigenetic bases, nanopore technology, and polymerase used.
Moreover, third-generation sequencing techniques, such as nanopore sequencing, allow for direct detection of modified bases (methylation) and structural variants in the genome. Traditional NGS methods rely on amplification steps that can introduce biases and errors into the data. In contrast, single-molecule sequencing using nanopore technology directly reads individual DNA molecules without the need for amplification. This approach provides a more accurate representation of the genome and allows researchers to detect epigenetic modifications (methylation) and structural variations with greater precision. The use of specialized polymerase enzymes further enhances the accuracy of this method.
By directly reading single DNA molecules, third-generation sequencing platforms such as nanopore and illumina offer unique insights into complex genomes like the human genome. The human genome contains numerous repetitive regions that are difficult to resolve using short-read NGS technologies due to their limited ability to span these regions entirely. However, with longer read lengths provided by third-generation platforms, scientists can overcome this challenge and obtain a more comprehensive understanding of these complex genomic structures through epigenetic bases and sequence analysis.
The use of nucleic acid-based polymerase molecules is another key feature of third-generation sequencing technology, also known as NGS technologies. These polymerases facilitate the incorporation of fluorescently labeled nucleotides during the sequencing process, allowing real-time monitoring of DNA synthesis. This real-time monitoring not only improves accuracy but also enables dynamic studies such as kinetic analysis of enzymatic reactions or tracking changes in gene expression over time. With the advancement of nanopore sequencing, methylation patterns can also be analyzed alongside the DNA sequence.
Applications of Next-Generation Sequencing in Genomics
Next-generation sequencing (NGS), including illumina and nanopore technologies, has revolutionized the field of genomics, offering unprecedented opportunities for scientific discoveries and medical advancements. The ability to sequence DNA and RNA at an unprecedented scale has transformed the way we study and understand the genetic makeup of organisms.
Whole-Genome Sequencing for Disease-Causing Mutations
One of the primary uses of next-generation sequencing (NGS) is in whole-genome sequencing, where it allows researchers to analyze an individual's entire DNA sequence. This approach, using technologies like Illumina and nanopore, has been instrumental in identifying disease-causing mutations and understanding their impact on human health. By comparing an individual's genome with a reference genome, scientists can identify genetic variations that may contribute to diseases such as cancer or rare genetic disorders.
Targeted Resequencing for Studying Specific Genes or Genomic Regions
Next generation sequencing (NGS) enables targeted resequencing of specific genes or genomic regions of interest. This approach is particularly useful when studying known disease-associated genes or investigating specific mutations within a population. By selectively sequencing these regions, researchers can gain valuable insights into the genetic basis of diseases and develop more targeted therapies. NGS is a powerful tool for analyzing the sequence of DNA molecules and understanding the generation DNA.
Metagenomic Studies Analyzing Microbial Communities
Another area where NGS shines is in metagenomic studies, allowing researchers to analyze microbial communities present in various environments using nanopore and illumina sequencing technologies. By sequencing DNA extracted from samples such as soil, water, or human gut microbiota, scientists can unravel the diversity and functional potential of microorganisms living within these ecosystems. This information contributes to our understanding of microbial ecology and aids in developing strategies for managing environmental issues or improving human health through the analysis of nucleotides.
Epigenetic Bases Expanding Molecular Biology Research
Epigenetics refers to changes in gene expression that are not caused by alterations in the underlying DNA sequence itself but rather modifications to the structure or packaging of DNA molecules. Next generation sequencing (NGS) plays a crucial role in studying epigenetic mechanisms by enabling the analysis of DNA methylation patterns, histone modifications, and chromatin accessibility. This information helps researchers unravel the complex regulatory networks that control gene expression and provides insights into various biological processes, including development, aging, and disease. NGS is particularly useful for studying RNA sequencing, cell sequencing, and whole genome sequencing.
NGS in Cancer Research
Cancer research has greatly benefited from next generation sequencing (NGS) technologies. By sequencing cancer genomes, scientists can identify genetic alterations driving tumor initiation and progression. This knowledge aids in developing personalized treatment strategies tailored to an individual's specific genomic profile. NGS facilitates the identification of novel therapeutic targets and biomarkers for early detection or monitoring treatment response. The use of Illumina technology enables efficient sequencing of the DNA molecule, providing valuable insights into the generation DNA.
Utilizing Third-Generation Sequencing for Structural Variant Detection
Third-generation sequencing, also known as NGS technologies, is a revolutionary NGS platform that has transformed the field of genomics. It allows researchers to accurately detect large-scale structural variations in the genome, providing invaluable insights into the complexity of genetic architecture. By leveraging this cutting-edge NGS technique, scientists can identify chromosomal rearrangements, inversions, deletions, and duplications with unprecedented precision at the sequence level of the DNA molecule.
One of the key advantages of third-generation sequencing (NGS technologies) is its ability to detect structural variants (DNA molecules) that were previously challenging to identify using traditional sequencing methods (SGS technologies). These structural variations (DNA molecules) play a crucial role in human health and disease, contributing to genetic disorders and influencing an individual's susceptibility to various conditions. With third-generation sequencing (NGS technologies), researchers can now delve deeper into the intricacies of these structural changes (DNA molecules) and gain a better understanding of their functional implications.
The detection capabilities offered by third-generation sequencing (NGS technologies) are particularly valuable when studying complex diseases with a strong genomic component. For instance, certain cancers exhibit extensive genomic rearrangements that drive tumor progression and therapy resistance. By employing third-generation sequencing (NGS technologies) techniques, scientists can comprehensively analyze these structural variants and uncover critical insights into cancer biology. This is because third-generation sequencing (NGS technologies) allows for the detailed sequencing of the DNA molecule, providing a more comprehensive view of the genetic alterations present in cancer cells compared to second-generation sequencing (SGS technologies).
To achieve accurate detection of structural variants using third-generation sequencing technologies, innovative approaches involving methylation and polymerase have been developed. One such method involves utilizing pyridine borane chemistry for library preparation. Pyridine borane efficiently converts DNA modifications induced by structural variations into detectable signals during sequencing using nanopore and NGS technologies. This technique has proven highly effective in enhancing the sensitivity and specificity of detecting large-scale genomic rearrangements.
Structural variant detection using third-generation sequencing involves several steps, including the analysis of the DNA sequence, the use of DNA polymerase to replicate the DNA molecule, and the identification of methylation patterns.
1. Library Preparation: The DNA sample undergoes fragmentation and adapter ligation to create a nanopore sequencing library suitable for single molecule methylation analysis.
2. Next generation sequencing: The DNA molecule library is loaded onto a sequencer capable of generating long reads using nanopore technology and DNA polymerase.
3. Data Analysis: Specialized bioinformatics tools are employed to align the long reads generated from cell sequencing, RNA sequencing, next generation sequencing, and nanopore sequencing to a reference genome and identify structural variations.
By following this workflow, researchers can obtain comprehensive and accurate information about structural variations present within the genome of interest using cell sequencing, epigenetic sequencing, and next generation sequencing. This next generation dna sequencing approach allows for a more in-depth analysis of the genome, providing valuable insights for research purposes.
The application of third-generation sequencing, such as SGS technologies, for structural variant detection in the DNA molecule has already yielded remarkable discoveries. For example, it has shed light on the role of large-scale chromosomal rearrangements, detected by DNA polymerase, in neurodevelopmental disorders like autism spectrum disorder. By unraveling these complex genomic changes, scientists are gaining insights into the underlying molecular mechanisms and potential therapeutic targets.
Next-Generation Sequencing for Disease Diagnosis
Next-generation sequencing (NGS), offered by SGS Technologies (SGS) and TGS, has revolutionized the field of disease diagnosis. It provides unprecedented insights into genetic disorders and enables more accurate and efficient detection of disease-causing mutations. NGS analyzes vast amounts of DNA sequence data rapidly, making it an invaluable tool in various diagnostic applications.
One area where next generation sequencing (NGS) excels is in identifying genetic abnormalities during prenatal testing. Non-invasive prenatal testing (NIPT) has been made possible through NGS, allowing healthcare professionals to detect fetal genetic abnormalities using maternal blood samples. This non-invasive approach eliminates the need for invasive procedures such as amniocentesis or chorionic villus sampling, reducing potential risks for both the mother and the fetus. By analyzing cell-free fetal DNA present in maternal blood, NGS can accurately identify chromosomal aberrations associated with conditions like Down syndrome, Edwards syndrome, and Patau syndrome. This demonstrates the power of epigenetic sequencing in enabling comprehensive whole genome sequencing. Additionally, emerging technologies like nanopore sequencing are further advancing the field of genetic analysis.
Cancer genomics greatly benefits from Next Generation Sequencing (NGS) technology. By sequencing cancer genomes, researchers can identify somatic mutations that play a crucial role in tumor development and progression. NGS allows for a comprehensive analysis of an individual's cancer genome, providing valuable information about the specific mutations driving the disease. This knowledge can guide personalized treatment strategies by identifying targetable mutations or predicting response to certain therapies. NGS is a powerful tool in cancer genomics.
In addition to identifying mutations directly related to diseases, next generation sequencing (NGS) also enables researchers to explore other aspects of genomic variation that may contribute to disease susceptibility or progression. For example, bisulfite sequencing can be used to assess DNA methylation status—a modification involved in gene regulation—providing insights into epigenetic changes associated with diseases such as cancer. NGS, also known as second-generation sequencing (SGS), has revolutionized the field of genomics and offers a powerful tool for studying genetic variations and their impact on health.
The advent of third-generation sequencing technologies like NovaSeq has further expanded the possibilities offered by NGS. These platforms offer longer read lengths and improved accuracy compared to previous generations, enabling de novo assembly of complex genomes and facilitating the identification of structural variations that may be missed by short-read sequencing. This enhanced capability is particularly valuable for unraveling the genetic basis of rare diseases or uncovering novel disease-causing mutations. With the use of DNA polymerase, these platforms can accurately sequence the DNA molecule, providing valuable insights into genetic variations and disease-causing mutations. The advanced technology of SGS allows for efficient and accurate sequencing, enabling researchers to uncover the sequence of the DNA molecule and identify any structural variations or mutations that may be present.
Next-generation sequencing (NGS) has also played a crucial role in infectious disease diagnostics, allowing for rapid and accurate identification of pathogens. By sequencing the DNA or RNA of microbial agents, NGS can provide valuable information about their genetic makeup, evolution, and drug resistance profiles. This knowledge aids in the development of targeted therapies, surveillance programs, and outbreak investigations. NGS is a powerful tool in the field of data analysis. It has paved the way for third generation sequencing (TGS), which further improves accuracy and efficiency in sequencing processes.
Leveraging Third-Generation Sequencing for Transcriptome Analysis
Third-generation sequencing, also known as TGS, has revolutionized the field of genomics by providing high-throughput, long-read capabilities that enable comprehensive transcriptome analysis. This advanced technology, which utilizes DNA polymerase, offers numerous advantages over previous generations, such as de novo assembly and SGS. It is a powerful tool for researchers in their quest to unravel the complexities of gene expression.
One of the key benefits of third-generation sequencing is its ability to identify alternative splicing events and novel transcripts. Alternative splicing plays a crucial role in expanding the proteomic diversity encoded by our genes. By accurately characterizing these alternative isoforms, researchers can gain deeper insights into cellular processes and disease mechanisms. With its long-read capabilities, third-generation sequencing enables the detection and characterization of complex splice variants that were previously challenging to capture using short-read technologies. This is possible due to the use of DNA polymerase, SGS, TGS, and other molecules.
Furthermore, third-generation sequencing (TGS) allows for isoform-level quantification of gene expression. Traditional methods often struggle with accurately quantifying gene expression due to their reliance on short reads that may not span across entire transcripts. In contrast, TGS generates longer reads that overcome this limitation, enabling more precise measurements of gene expression at an isoform level. This information is invaluable when studying complex biological systems where different isoforms play distinct roles. TGS is a powerful tool for understanding the complexities of DNA and de novo assembly, and it surpasses the limitations of short-read sequencing (SGS).
To leverage the power of third-generation sequencing (TGS) for transcriptome analysis, several steps need to be followed. The process begins with library preparation, where RNA molecules are converted into cDNA libraries suitable for sequencing. Various protocols exist for library preparation depending on the specific research goals and sample types. These protocols can be found through data sources such as SGS, ScholarCrossRefSearch, ADS, PubMed, and WorldCat.
Once the libraries of molecules are prepared, they undergo emulsion PCR (polymerase chain reaction), which amplifies DNA fragments within tiny water-in-oil droplets called emulsions. This step ensures sufficient DNA material for subsequent sequencing steps in generation NGS technologies. The data obtained from this process can include 5hmc information.
Next comes the actual sequencing process using third-generation platforms such as PacBio or Oxford Nanopore Technologies. These platforms generate raw data in the form of long reads spanning thousands of bases, which requires further processing and analysis to extract meaningful information about the DNA molecule.
DNA data analysis for third-generation sequencing (TGS) involves several steps. First, the raw data undergoes quality control to remove any low-quality reads or artifacts. Then, de novo assembly is performed to reconstruct the transcriptome from these long reads without relying on a reference genome. This approach is particularly useful when studying non-model organisms or when a reference genome is unavailable. The resulting data can be analyzed using specialized software and tools, such as the Sequence Generation System (SGS). To ensure proper citation and access to the research, researchers can assign a Digital Object Identifier (DOI) to their data.
Once the assembly is complete, researchers can perform downstream analyses such as differential gene expression and functional annotation using epigenetic sequencing, whole genome sequencing, next generation sequencing, and RNA sequencing. These analyses provide insights into the biological processes and pathways affected by changes in gene expression patterns.
In addition to transcriptome analysis, third-generation sequencing (TGS) has also found applications in other areas of genomics research. For example, it can be used for whole-genome sequencing, detecting DNA modifications (such as bisulfite sequencing for studying DNA methylation), and characterizing oxidized derivatives in various contexts. TGS, also known as single-molecule real-time sequencing (SMRT), offers significant advantages over second-generation sequencing (SGS) technologies. It enables long-read sequencing, reducing the need for assembly and improving genome coverage. TGS platforms, like the ones offered by ScholarCrossrefSearch Ads PubmedWorldcat, have revolutionized genomics research.
Future Perspectives of Next-Generation and Third-Generation Sequencing
NGS evolution: accuracy, cost reduction, and faster turnaround times
Next-generation sequencing (NGS) has revolutionized the field of genomics, enabling researchers to decipher the complex genetic code with unprecedented speed and accuracy. As technology advances, NGS is expected to continue evolving, bringing forth improvements in several key areas such as DNA sequencing, SGS (second-generation sequencing), TGS (third-generation sequencing), and the analysis of DNA molecules.
One major area of focus is enhancing the accuracy of DNA sequencing results. While current NGS platforms already offer impressive precision, future iterations, such as third generation sequencing (TGS) and single molecule sequencing (SGS), are anticipated to further refine this aspect. This increased accuracy will enable scientists to confidently identify even the subtlest variations in the genome, shedding light on previously undetected genetic factors that contribute to various diseases.
Another crucial aspect that is poised for improvement is cost reduction in DNA sequencing. Currently, NGS (next-generation sequencing) can be quite expensive due to the sophisticated equipment and reagents involved. However, ongoing research and development efforts aim to streamline processes and optimize workflows, ultimately driving down costs associated with DNA sequencing. This cost reduction will make genomic analysis more accessible to a wider range of researchers and healthcare professionals interested in SGS (second-generation sequencing) and TGS (third-generation sequencing).
There is a growing demand for faster turnaround times in genomic studies. Researchers often require timely results in order to make informed decisions or provide prompt diagnoses in clinical settings. To address this need, future advancements in DNA sequencing (NGS) are expected to significantly reduce the time required for sample preparation, sequencing runs, data analysis, and result interpretation. Ultimately, this accelerated process will expedite scientific discoveries and improve patient care outcomes. With the advent of third generation sequencing (TGS), the time taken for NGS will be further reduced, meeting the demands of researchers and clinicians alike.
Third-generation sequencing technologies: longer read lengths and increased throughput
While next-generation sequencing (NGS) has been groundbreaking in its own right, third-generation sequencing (TGS) technologies hold immense promise for further advancements in genomics research. One area where these technologies are anticipated to excel is read length, allowing for more comprehensive analysis of DNA molecules.
Current NGS platforms, such as SGS, typically generate short reads of DNA fragments during sequencing processes. In contrast, third-generation sequencing techniques, like PG, have the potential to produce much longer reads spanning entire genomic regions. Longer read lengths enable researchers to overcome challenges posed by repetitive sequences and complex structural variants, allowing for a more comprehensive understanding of the genome. This is beneficial for analyzing and interpreting data obtained from molecular studies.
In addition to longer read lengths, third-generation DNA sequencing technologies, such as SGS, are expected to increase throughput. Greater throughput means that larger volumes of data can be generated in a shorter period of time, facilitating large-scale genomic studies and accelerating scientific breakthroughs. This advancement in DNA sequencing technology is crucial for researchers and scientists, as it enables them to obtain more accurate and comprehensive information about the molecule of life. To learn more about this topic, please refer to the DOI provided.
Integration of NGS and third-generation sequencing: a comprehensive understanding of the genome
The integration of next-generation sequencing (NGS) and third-generation sequencing (TGS) holds immense potential in unlocking a deeper understanding of the genome. By combining the strengths of both technologies, researchers can achieve a more comprehensive picture of genetic variations and their functional implications. This integration allows for the analysis of DNA molecules and the generation of vast amounts of data.
Next-generation sequencing (NGS) provides high accuracy, cost-effectiveness, and faster turnaround times for large-scale genomic studies involving numerous DNA samples. On the other hand, third-generation sequencing (TGS) brings longer read lengths and increased throughput capabilities, enabling researchers to explore previously inaccessible regions of the genome with greater detail and obtain more comprehensive data.
This integration allows scientists to overcome limitations associated with each technology individually, including whole genome sequencing, next generation sequencing, and epigenetic sequencing. By leveraging both approaches simultaneously, researchers can obtain a holistic view of genetic variations across various scales – from single nucleotide changes to large structural rearrangements. This deeper understanding will facilitate advancements in personalized medicine, disease diagnostics, and targeted therapies using the latest generation NGS technologies.
Future Perspectives of Next-Generation and Third-Generation Sequencing Technologies
Advancements in bioinformatics tools will facilitate the analysis of large-scale genomic data generated by DNA sequencing techniques such as NGS and third-generation sequencing. With the help of SGS, scholarcrossrefsearch ads pubmedworldcat, researchers can efficiently analyze the vast amount of data obtained from these sequencing methods. This will enable them to gain valuable insights into the complex nature of genetic information.
The field of genomics has witnessed remarkable progress with the advent of next-generation sequencing (NGS) technologies. These cutting-edge techniques have revolutionized DNA sequencing, enabling researchers to obtain vast amounts of genetic information quickly and at a lower cost compared to traditional methods. However, as technology continues to evolve, the potential for even more significant advancements becomes evident. To access scholarly articles related to genomics research, you can use platforms like Google Scholar, CrossRef, PubMed, and WorldCat. Additionally, you can find relevant datasets on platforms like BASE. If you are looking for a specific article, make sure to search for its DOI or use the page number (pg) for easy navigation.
One crucial aspect that will shape the future of NGS and third-generation sequencing is the development of advanced bioinformatics tools. With the massive amount of genomic data being generated, efficient analysis is paramount. Bioinformatics algorithms, such as scholarcrossrefsearch, ads, pubmedworldcat, are continuously being refined to handle big data sets, ensuring accurate identification and interpretation of genetic variations at the dna level.
Artificial intelligence (AI) algorithms are expected to play a vital role in improving variant calling accuracy and interpretation in next generation sequencing (NGS), epigenetic sequencing, RNA sequencing, and DNA. Machine learning models can be trained on vast databases of genetic information, allowing them to identify patterns and predict variants with higher precision than ever before. By integrating AI into NGS workflows, researchers can streamline their analyses while maintaining high-quality results.
Long-read DNA sequencing, offered by third-generation technologies, has the potential to revolutionize genomics research. With the help of doi, scholarcrossrefsearch ads pubmedworldcat, researchers can access a vast database of information. This technology allows for the analysis of longer DNA fragments, providing more comprehensive insights into genetic variations and structural rearrangements. As a result, genomics research can advance significantly, leading to breakthrough discoveries and advancements in various fields such as pg and de.
While NGS (Next-Generation Sequencing) has been instrumental in advancing our understanding of genetics, it does have limitations. This is where third-generation sequencing technologies, such as DNA sequencing, come into play. These novel approaches offer long-read capabilities that allow scientists to sequence much larger fragments or even whole genomes in a single read. These advancements have been supported by scholarly resources like PubMed, WorldCat, and ScholarCrossRefSearch Ads.
The potential impact of long-read DNA sequencing on studying complex genomic regions such as repetitive sequences or structural variations cannot be overstated. It opens up new avenues for accurate analysis using current generation sequencers. Moreover, this technology holds great promise for unraveling intricate aspects like epigenetic modifications and genome assembly. With pg, vol, scholarcrossrefsearch ads, de, the potential of this technology is limitless.
Unlocking the Potential of Third-Generation Sequencing for Longread DNA Sequencing
Third-generation DNA sequencing platforms have revolutionized the field of genomics by enabling the generation of long reads, surpassing the limitations associated with short-read technologies. This breakthrough has opened up new possibilities and paved the way for a deeper understanding of complex genomic regions that were previously challenging to analyze. The use of these platforms has allowed for the analysis of DNA at the base level, providing valuable insights into the structure and function of genetic material.
One significant advantage of long-read DNA sequencing, also known as next-generation sequencing (NGS) technologies, is its ability to facilitate de novo assembly. Unlike short reads, which often require extensive computational effort to piece together, long reads provide a more complete picture of the genome. With longer read lengths, researchers can confidently resolve structural variations, repetitive sequences, and haplotype phasing with greater accuracy. This is why long-read sequencing has become increasingly popular in the scientific community.
The increased sequencing depth achieved through third-generation sequencing allows for a more comprehensive analysis of the whole genome. Short-read technologies often struggle to capture certain genomic regions due to their limited read lengths. In contrast, long reads provide a more thorough coverage of DNA, ensuring that no crucial information is missed during analysis. The longer read lengths in third-generation sequencing allow for a higher volume of base pairs to be captured, resulting in a more detailed and accurate understanding of the genome.
To achieve these impressive results, third-generation sequencing platforms utilize innovative techniques such as polymerase kinetics and direct methylation detection. Polymerase kinetics enable real-time monitoring of DNA synthesis by measuring changes in electrical current as nucleotides are incorporated into growing strands. This approach not only provides accurate base reads but also allows for detection of modifications such as methylations. With the incorporation of these techniques, the vol of accurate base reads and detection of modifications is achieved.
Another key component in third-generation sequencing is the use of motor proteins and nanopore technology. Motor proteins control the movement of DNA strands through nanopores embedded in specialized membranes, providing a solid base for sequencing. As each nucleotide passes through the pore, it generates unique electrical signals that can be decoded into sequence information. This method eliminates the need for PCR amplification and significantly reduces bias introduced during sample preparation. With scholarcrossrefsearch ads, researchers can easily access relevant scholarly articles to enhance their understanding of this cutting-edge technology.
Long-read DNA sequencing, also known as next-generation sequencing (NGS), has already demonstrated its potential across various fields of study. For example, scholars are utilizing NGS to search for relevant articles using ScholarCrossrefSearch Ads. This technology allows for the sequencing of longer stretches of DNA bases, providing a more comprehensive understanding of genetic information.
· In cancer research, long-read sequencing has revealed complex structural rearrangements within tumor genomes that were previously undetectable with short-read technologies. This advancement in DNA analysis using long-read sequencing, also known as next-generation sequencing (NGS), has allowed researchers to uncover hidden genetic information. With the help of ScholarCrossRefSearch ads, scientists can now access a vast volume of scholarly articles and publications on this topic.
· In evolutionary biology, long reads have shed light on the dynamics of repetitive sequences and transposable elements, providing insights into genome evolution. Additionally, epigenetic sequencing and next generation sequencing techniques have been used to analyze DNA and RNA sequencing data.
· In microbiology, long-read sequencing has enabled the characterization of complex microbial communities and the identification of novel species using DNA. With the advancements in next generation sequencing (NGS), scholars can now easily access relevant research articles through ScholarCrossrefSearch ADS. This technology has revolutionized the field by providing a wealth of information and insights into microbial diversity and evolution.
Concluding Insights on the Capabilities of Next-Generation and Third-Generation Sequencing
In conclusion, both next-generation sequencing (NGS) and third-generation sequencing technologies have revolutionized genomics research by enabling unprecedented insights into the genetic makeup of organisms. NGS, also known as DNA sequencing, has brought about significant advancements in genomics through its ability to generate vast amounts of data quickly and cost-effectively. This technology has found extensive applications in various fields, including disease diagnosis, structural variant detection, and transcriptome analysis. ScholarCrossrefSearch Ads can help researchers find relevant scholarly articles related to these advancements in sequencing technologies.
On the other hand, third-generation sequencing has emerged as a promising technology for long-read DNA sequencing, offering the potential to unlock new frontiers in genomics research. By providing longer reads that can span complex genomic regions, this technology is particularly valuable for detecting structural variations and understanding gene expression patterns. With its capabilities, third-generation sequencing is revolutionizing the field of genomics.
Looking ahead, the future perspectives of both DNA sequencing technologies, including next-generation and third-generation sequencing, hold immense promise. Next-generation sequencing technologies, such as scholarcrossrefsearch ads, are constantly evolving to improve read lengths, reduce costs, and enhance accuracy. These advancements will further expand the applications of NGS in diverse areas such as personalized medicine, agriculture, and environmental studies.
Similarly, third-generation DNA sequencing technologies, such as scholarcrossrefsearch ads, are expected to continue advancing rapidly. Efforts are underway to overcome challenges related to error rates and throughput limitations associated with this vol technology. As these hurdles are overcome, we can anticipate even greater utilization of third-generation sequencing for comprehensive genome analysis.
To fully leverage the potential of next-generation and third-generation sequencing technologies, researchers and clinicians must stay updated with the latest developments in this field. By embracing these cutting-edge tools, we can unravel intricate genetic mechanisms underlying diseases, develop targeted therapies based on individual genomic profiles, and make significant strides towards precision medicine. This includes utilizing DNA and ScholarCrossrefSearch Ads to access relevant scholarly articles and stay informed about the latest advancements in the field. Keeping up-to-date with the latest research in this vol field is crucial for progress in precision medicine.
In summary, next-generation sequencing, including scholarcrossrefsearch ads, has become an indispensable tool for genomics research due to its speed, cost-effectiveness, and wide-ranging applications. Simultaneously, third-generation sequencing is poised to transform long-read DNA sequencing with its ability to provide more comprehensive genomic information. By harnessing the power of both technologies, we can unravel the complexities of the genome and pave the way for a new era of personalized medicine.
FAQs
Q: How does next-generation sequencing contribute to disease diagnosis?
Next-generation sequencing plays a crucial role in disease diagnosis by enabling comprehensive analysis of an individual's DNA. It allows for the identification of genetic variants associated with diseases, aiding in accurate diagnosis and personalized treatment strategies. The use of this technology has revolutionized the field of genetics, providing valuable insights into an individual's vol profile.
Q: Can third-generation sequencing detect structural variations in the genome?
Yes, third-generation DNA sequencing is particularly well-suited for detecting structural variations due to its ability to generate long reads that span complex genomic regions. This technology provides valuable insights into large-scale genomic rearrangements and contributes to understanding genetic diversity among individuals. The use of this technology helps in the identification of vol differences in the DNA.
Q: What are some potential applications of next-generation sequencing beyond genomics research?
Next-generation sequencing has diverse applications beyond genomics research. It is used in fields such as forensic science, evolutionary biology, metagenomics (study of microbial communities), and ancient DNA analysis. Its versatility makes it a powerful tool across various scientific disciplines.
Q: How can researchers stay updated with advancements in next-generation and third-generation sequencing technologies?
To stay updated with advancements in generation NGS and DNA sequencing, researchers can regularly attend conferences, workshops, and webinars focused on genomics and sequencing. They can also follow reputable scientific journals, online forums, and social media platforms dedicated to genomics research. Keeping up with the latest developments in generation NGS and DNA sequencing is crucial for researchers in this field.
Q: Are there any limitations or challenges associated with third-generation sequencing?
Third-generation DNA sequencing still faces challenges related to error rates and throughput limitations compared to other sequencing methods. However, ongoing efforts are addressing these limitations through technological innovations and improvements in data analysis algorithms. The advancements in DNA sequencing technology aim to improve the accuracy and efficiency of vol sequencing.
What's Your Reaction?
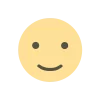
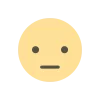
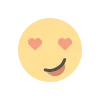
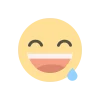
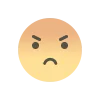
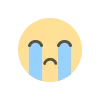
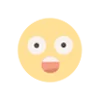